by Nicola Phillips, Copywriter
When an electrical signal flows through a wire, it creates a corresponding magnetic field. The flow of energy contained in that field is called reactive power.
Energy moves via currents. The U.S. electrical grids, like most electrical grids, run on alternating current (AC), as opposed to direct current (DC). Direct current is kind of like tennis balls contained in a tube. When you push a new ball into the tube, the force of the new object in the confined space causes the ball at the other end to pop out. The energy generated by the first ball moves in a straight line at a steady level. Electronics for Dummies likens alternating current to the metal balls in the game Newton’s Cradle. Pulling the ball at one end and then releasing it causes the first ball to hit the next one in the series, and immediately upon impact the ball farthest away from the first one swings away from the group.
In AC circuits, current periodically bounces, refracts, changes direction, and alternates voltage. The volatility of AC circuits is actually an advantage in grid systems, as it allows voltages to easily move between higher and lower levels (higher voltages are easier to transfer across large distances).
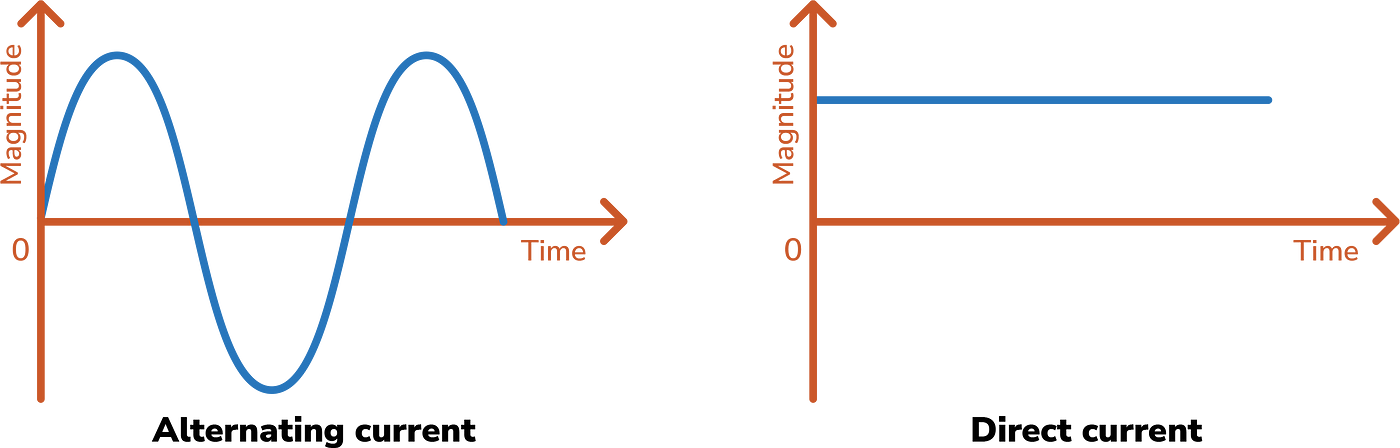
When current alternates, some of the input energy gets stored in the circuit inductors’ magnetic field. During the next current cycle, that energy gets bounced back to the source and never makes it to the load. That’s reactive power. The springiness of AC circuits creates the conditions for reactive power to exist.
Effective, or active, power is what makes it to your load. Reactive power doesn’t actually do any work, which is why it’s sometimes referred to as “imaginary power,” and loss of energy in a load due to reactive power can be significant. Apparent power (the total amount of power that enters a circuit) does not exactly translate to active power (the power that actually makes it to the load) because of the presence of reactive power in a circuit.

Reactive power impacts a system’s power factor, the ratio of working power, measured as:
kVA = V x A
(the ratio of active power to total apparent power)
More reactive power decreases system power factor, which in turn contributes to conduction losses, excess heating, unwanted voltage drops, lower operational efficiency, and higher operational costs.
A bad power factor contributes to a high Power Usage Effectiveness (PUE), another measure of data center efficiency. High PUE is a signal of wasted energy, and it translates to inefficient operations.
In both AC and DC circuits, there is resistance — voltage divided by current — that impedes the flow of current. In AC circuits, there is also reactance, which opposes changes in current. Reactance is determined by frequency, and exists in both inductors and capacitors.
In a circuit that has both resistance and reactance, some power will be dissipated and some will be absorbed and returned. Some power will be active and some will be reactive. Voltage and current in such a circuit will be out of phase by a value somewhere between 0° and 90°.
Journey from the grid to a Soluna MDC
To get to one of our MDCs, energy travels from the grid, where it has a voltage of 345 kV, to a substation, where its voltage changes to 34.5 kV. It moves from the substation to a switchgear and then to a transformer at the same voltage. The transformer changes the alternating current into a direct current with a voltage of 416 V for three-phase or 240 V for single phase. The current moves through another switchgear and arrives at the Soluna MDCs.
A simplified way to think of the relationship between energy’s journey and reactive load is that every time you add a step from the substation, you create more reactive load.
Controlling reactive load on site
Fans, motors, and compressors are all things that use alternating current to run, and each causes springiness and resultant reactive load.
Crypto miners have four major components: a hash board, fans, a control board, and a power supply (PSU). A PSU is essentially the data center equivalent of the brick that connects your laptop to a power outlet. Each PSU plugs into a PDU, which is like a power strip. In Soluna data centers, every one of our miners has a PSU sitting in front of it to block the springiness of the fans.
The huge fans that sit outside of our MDCs can also cause springiness, but they are behind what’s called a VFD (variable frequency drive), a device that lets us control the speed of the fan and also blocks the springiness.
Air conditioners, pumps, plumbing for water — all of the things that we don’t have in our MDCs — generate lots of reactive load. The small amount of reactive load we do produce on our sites is mostly due to the transformation of power from one voltage to another in the transformers.
A lot of reactive power on a site, like a high PUE, is a sign of deeper inefficiencies in a power system. At Soluna, we’re designing sites that maximize the amount of power that actually makes it to its end destination — in this case, high-performance computing data centers. Check out what we’re working on.